Axis Formation in Amphibians_ The Pheno
.pdf
keyboard_arrow_up
School
Johns Hopkins University *
*We aren’t endorsed by this school
Course
020.363
Subject
Biology
Date
Oct 30, 2023
Type
Pages
15
Uploaded by DeanNeutronHedgehog15
NCBI Bookshelf. A service of the National Library of Medicine, National Institutes of Health.
Gilbert SF. Developmental Biology. 6th edition. Sunderland (MA): Sinauer Associates; 2000.
Axis Formation in Amphibians: The Phenomenon of the Organizer
The Progressive Determination of the Amphibian Axes
Vertebrate axes do not form from localized determinants in the various blastomeres, as in
Drosophila.
Rather, they arise progressively through a sequence of interactions between neighboring cells.
Amphibian axis formation is an example of regulative development. In Chapter 3, we discussed the
concept of regulative development, wherein (1) an isolated blastomere has a potency greater than its
normal embryonic fate, and (2) a cell's fate is determined by interactions between neighboring cells.
Such interactions are called inductions (see Chapter 6). That such inductive interactions were
responsible for amphibian axis determination was demonstrated by the laboratory of Hans Spemann at
the University of Freiburg. The experiments of Spemann and his students framed the questions that
experimental embryologists asked for most of the twentieth century, and they resulted in a Nobel Prize
for Spemann in 1935. More recently, the discoveries of the molecules associated with these inductive
processes have provided some of the most exciting moments in contemporary science.
The experiment that began this research program was performed in 1903, when Spemann demonstrated
that early newt blastomeres have identical nuclei, each capable of producing an entire larva. His
procedure was ingenious: Shortly after fertilizing a newt egg, Spemann used a baby's hair taken from
his daughter to lasso the zygote in the plane of the first cleavage. He then partially constricted the egg,
causing all the nuclear divisions to remain on one side of the constriction. Eventually, often as late as
the 16-cell stage, a nucleus would escape across the constriction into the non-nucleated side. Cleavage
then began on this side, too, whereupon Spemann tightened the lasso until the two halves were
completely separated. Twin larvae developed, one slightly older than the other (
Figure 10.17). Spemann
concluded from this experiment that early amphibian nuclei were genetically identical and that each
cell was capable of giving rise to an entire organism.
Figure 10.17
Spemann's demonstration of nuclear equivalence in newt cleavage.
(A) When the fertilized egg of the newt
Triturus taeniatus
was
constricted by a ligature, the nucleus was restricted to one-half of
the embryo. The cleavage on that side of the embryo reached
(more...)
However, when Spemann performed a similar experiment with the constriction still longitudinal, but
perpendicular to the plane of the first cleavage (separating the future dorsal and ventral regions rather
than the right and left sides), he obtained a different result altogether. The nuclei continued to divide on
both sides of the constriction, but only one side—the future dorsal side of the embryo—gave rise to a
normal larva. The other side produced an unorganized tissue mass of ventral cells, which Spemann
called the
Bauchstück
—the belly piece. This tissue mass was a ball of epidermal cells (ectoderm)
containing blood and mesenchyme (mesoderm) and gut cells (endoderm), but no dorsal structures such
as nervous system, notochord, or somites (
Figure 10.18).
Figure 10.18
Asymmetry in the amphibian egg. (A) When the egg is divided
along the plane of first cleavage into two blastomeres, each of
which gets one-half of the gray crescent, each experimentally
separated cell develops into a normal embryo. (B) When only one
of
(more...)
Why should these two experiments give different results? One possibility was that when the egg was
divided perpendicular to the first cleavage plane, some
cytoplasmic
substance was not equally
distributed into the two halves. Fortunately, the salamander egg was a good place to test that
hypothesis. As we have seen in Chapter 7 and above, there are dramatic movements in the cytoplasm
following the fertilization of amphibian eggs, and in some amphibians these movements expose a gray,
crescent-shaped area of cytoplasm in the region directly opposite the point of sperm entry. This area has
been called the
gray crescent.
Moreover, the first cleavage plane normally splits the gray crescent
equally into the two blastomeres. If these cells are then separated, two complete larvae develop.
However, should this cleavage plane be aberrant (either in the rare natural event or in an experiment),
the gray crescent material passes into only one of the two blastomeres. Spemann found that when these
two blastomeres are separated, only the blastomere containing the gray crescent develops normally.
WEBSITE
10.3 Embryology and individuality.
One egg usually makes only one adult. However, there are
exceptions to this rule, and Spemann was drawn into embryology through the paradoxes of
creating more than one individual from a single egg.
http://www.devbio.com/chap10/link1003.shtml
It appeared, then, that something in the gray crescent region was essential for proper embryonic
development. But how did it function? What role did it play in normal development? The most
important clue came from the fate map of this area of the egg, for it showed that the gray crescent
region gives rise to the cells that initiate gastrulation. These cells form the dorsal lip of the blastopore.
The cells of the dorsal lip are committed to invaginate into the blastula, thus initiating gastrulation and
the formation of the notochord. Because all future amphibian development depends on the interaction
of cells rearranged during gastrulation, Spemann speculated that the importance of the gray crescent
material lies in its ability to initiate gastrulation, and that crucial developmental changes occur during
gastrulation.
In 1918, Spemann demonstrated that enormous changes in cell potency do indeed take place during
gastrulation. He found that the cells of the
early
gastrula were uncommitted, but that the fates of
late
gastrula cells were determined. Spemann demonstrated this by exchanging tissues between the
gastrulae of two species of newts whose embryos were differently pigmented (
Figure 10.19). When a
region of prospective epidermal cells from an
early
gastrula was transplanted into an area in another
early gastrula where the neural tissue normally formed, the transplanted cells gave rise to neural tissue.
When prospective neural tissue from early gastrulae was transplanted to the region fated to become
belly skin, the neural tissue became epidermal (
Table 10.1). Thus, these early newt gastrula cells were
not yet committed to a specific fate. Such cells are said to exhibit
conditional
(i.e., regulative or
dependent)
development
because their ultimate fates depend on their location in the embryo. However,
when the same interspecies transplantation experiments were performed on
late
gastrulae, Spemann
obtained completely different results. Rather than differentiating in accordance with their new location,
the transplanted cells exhibited
autonomous
(or independent, or mosaic)
development
. Their
prospective fate was determined, and the cells developed independently of their new embryonic
location. Specifically, prospective neural cells now developed into brain tissue even when placed in the
region of prospective epidermis, and prospective epidermis formed skin even in the region of the
prospective neural tube. Within the time separating early and late gastrulation, the potencies of these
groups of cells had become restricted to their eventual paths of differentiation. Something was causing
them to become determined to epidermal and neural fates. What was happening?
Figure 10.19
Determination of ectoderm during newt gastrulation. Presumptive
neural ectoderm from one newt embryo is transplanted into a
region in another embryo that normally becomes epidermis. (A)
When the tissues are transferred between early gastrulas, the
presumptive
(more...)
Table 10.1
Results of tissue transplantation during early- and late-gastrula
stages in the newt.
Hans Spemann and Hilde Mangold: Primary Embryonic Induction
The most spectacular transplantation experiments were published by Hans Spemann and Hilde
Mangold in 1924.
They showed that, of all the tissues in the early gastrula, only one has its fate
determined. This self-differentiating tissue is the dorsal lip of the blastopore, the tissue derived from the
gray crescent cytoplasm. When this dorsal lip tissue was transplanted into the presumptive belly skin
region of another gastrula, it not only continued to be blastopore lip, but also initiated gastrulation and
embryogenesis in the surrounding tissue (
Figure 10.20). Two conjoined embryos were formed instead
of one!
Figure 10.20
Organization of a secondary axis by dorsal blastopore lip tissue.
(A) Dorsal lip tissue from an early gastrula is transplanted into
another early gastrula in the region that normally becomes ventral
epidermis. (B) The donor tissue invaginates and forms
(more...)
In these experiments, Spemann and Mangold used differently pigmented embryos from two newt
species: the darkly pigmented
Triturus taeniatus
and the nonpigmented
Triturus cristatus.
So when
Spemann and Mangold prepared these transplants, they were able to readily identify host and donor
tissues on the basis of color.
When the dorsal lip of an early
T. taeniatus
gastrula was removed and
implanted into the region of an early
T. cristatus
gastrula fated to become ventral epidermis (belly
skin), the dorsal lip tissue invaginated just as it would normally have done (showing self-
determination), and disappeared beneath the vegetal cells. The pigmented donor tissue then continued
to self-differentiate into the chordamesoderm (notochord) and other mesodermal structures that
normally form from the dorsal lip. As the new donor-derived mesodermal cells moved forward, host
cells began to participate in the production of the new embryo, becoming organs that normally they
*
†
never would have formed. In this secondary embryo, a somite could be seen containing both pigmented
(donor) and unpigmented (host) tissue. Even more spectacularly, the dorsal lip cells were able to
interact with the host tissues to form a complete neural plate from host ectoderm. Eventually, a
secondary embryo formed, face to face with its host. These technically difficult experiments have been
repeated using nuclear markers, and the results of Spemann and Mangold have been confirmed (
Smith
and Slack 1983;
Recanzone and Harris 1985).
WEBSITE
10.4 Spemann, Mangold, and the organizer.
Spemann did not see the importance of this work
the first time they did it. This website provides a more detailed account of why Spemann and
Mangold did this experiment.
http://www.devbio.com/chap10/link1004.shtml
Spemann (1938) referred to the dorsal lip cells and their derivatives (notochord, prechordal mesoderm)
as the
organizer
because (1) they induced the host's ventral tissues to change their fates to form a
neural tube and dorsal mesodermal tissue (such as somites), and (2) they organized host and donor
tissues into a secondary embryo with clear anterior-posterior and dorsal-ventral axes. He proposed that
during normal development, these cells organize the dorsal ectoderm into a neural tube and transform
the flanking mesoderm into the anterior-posterior body axis. It is now known (thanks largely to
Spemann and his students) that the interaction of the chordamesoderm and ectoderm is not sufficient to
“organize” the entire embryo. Rather, it initiates a series of sequential inductive events. As discussed in
Chapter 6, the process by which one embryonic region interacts with a second region to influence that
second region's differentiation or behavior is called induction. Because there are numerous inductions
during embryonic development, this key induction wherein the progeny of dorsal lip cells induce the
dorsal axis and the neural tube is traditionally called
primary embryonic induction
.
The Mechanisms of Axis Formation in Amphibians
The experiments of Spemann and Mangold showed that the dorsal lip of the blastopore, and the
notochord that forms from it, constituted an “organizer” that could instruct the formation of new
embryonic axes. But the mechanisms by which the organizer was constructed and through which it
operated were totally unknown. Indeed, it is said that Spemann and Mangold's paper posed more
questions than it answered. Among these questions were:
How did the organizer get its properties? What caused the dorsal blastopore lip to differ from
any other region of the embryo?
What factors were being secreted from the organizer to cause the formation of the neural tube
and to create the anterior-posterior, dorsal-ventral, and left-right axes?
How did the different parts of the neural tube become established, with the most anterior
becoming the sensory organs and forebrain, and the most posterior becoming spinal cord?
We will take up each of these questions in turn.
The origin of the Nieuwkoop center
The major clue in determining how the dorsal blastopore lip obtained its properties came from the
experiments of
Pieter Nieuwkoop (1969,
1973,
1977). He and his colleagues in the Netherlands
‡
demonstrated that the properties of this newly formed mesoderm were induced by the vegetal
(presumptive endoderm) cells underlying them. He removed the equatorial cells (i.e., presumptive
mesoderm) from a blastula and showed that neither the animal cap (presumptive ectoderm) nor the
vegetal cap (presumptive endoderm) produced any mesodermal tissue. However, when the two caps
were recombined, the animal cap cells were induced to form mesodermal structures such as notochord,
muscles, kidney cells, and blood cells (
Figure 10.21). The polarity of this induction (whether the animal
cells formed dorsal mesoderm or ventral mesoderm) depended on the dorsal-ventral polarity of the
endodermal (vegetal) fragment. While the ventral and lateral vegetal cells (those closer to the side of
sperm entry) induced ventral (mesenchyme, blood) and intermediate (muscle, kidney) mesoderm, the
dorsalmost vegetal cells specified dorsal mesoderm components (somites, notochord), including those
having the properties of the organizer. The dorsalmost vegetal cells of the blastula, which are capable of
inducing the organizer, have been called the
Nieuwkoop center
(
Gerhart et al. 1989).
Figure 10.21
Summary of experiments by Nieuwkoop and by Nakamura and
Takasaki (1970), showing mesodermal induction by vegetal
endoderm. (A) Isolated animal cap cells become a mass of ciliated
epidermis, isolated vegetal cells generate gutlike tissue, and
isolated
(more...)
The Nieuwkoop center was demonstrated in the 32-cell
Xenopus
embryo by transplantation and
recombination experiments. First, Gimlich and Gerhart (
Gimlich and Gerhart 1984;
Gimlich 1985,
1986) performed an experiment analogous to the Spemann and Mangold studies, except that they used
blastulae rather than gastrulae. When they transplanted the dorsalmost vegetal blastomere from one
blastula into the ventral vegetal side of another blastula, two embryonic axes were formed (see
Figure
10.11B). Second,
Dale and Slack (1987) recombined single vegetal blastomeres from a 32-cell
Xenopus
embryo with the uppermost animal tier of a fluorescently labeled embryo of the same stage.
The dorsalmost vegetal cell, as expected, induced the animal pole cells to become dorsal mesoderm.
The remaining vegetal cells usually induced the animal cells to produce either intermediate or ventral
mesodermal tissues (
Figure 10.22). Thus, dorsal vegetal cells can induce animal cells to become dorsal
mesodermal tissue.
Figure 10.22
The regional specificity of mesoderm induction can be
demonstrated by recombining cells of 32-cell
Xenopus
embryos.
Animal pole cells were labeled with fluorescent polymers so that
their descendants could be identified, then combined with
individual vegetal
(more...)
The Nieuwkoop center is created by the cytoplasmic rotation that occurs during fertilization (see
Chapter 7). When this rotation is inhibited by UV light, the resulting embryo will not form dorsal-
anterior structures such as the head or neural tube (
Vincent and Gerhart 1987). However, these UV-
treated embryos can be rescued by transplantation of the dorsalmost vegetal blastomeres from a normal
embryo at the 32-cell stage (
Dale and Slack 1987; see
Figure 10.11A). If eggs are rotated toward the
end of the first cell cycle so that the future ventral side is upward, two Nieuwkoop centers are formed,
leading to two dorsal blastopore lips and two embryonic axes (see
Figure 10.10). Therefore, the
specification of the dorsal-ventral axis begins at the moment of sperm entry.
The molecular biology of the Nieuwkoop center
In
Xenopus
,
the endoderm is able to induce the formation of mesoderm by causing the presumptive
mesodermal cells to express the
Xenopus
Brachyury
(
Xbra
) gene. The mechanism of this induction is
not well understood (see
Harland and Gerhart 1997), but the Xbra protein is a transcription factor that
activates the genes that produce mesoderm-specific proteins. While all the vegetal cells appear to be
able to induce the overlying marginal cells to become mesoderm, only the dorsalmost vegetal cells can
instruct the overlying dorsal marginal cells to become the organizer. The major candidate for the factor
that forms the Nieuwkoop center in these dorsalmost vegetal cells is
β-catenin.
WEBSITE
10.5 Mesoderm induction.
There are numerous theories concerning how the generic mesoderm is
induced by the endoderm. Evidence points to three molecules as possible mesoderm inducers:
bFGF, Vg1, and an activin-like protein. These proteins can activate
Xbra
as well as other
mesodermal proteins.
http://www.devbio.com/chap10/link1005.shtml
β-catenin is a multifunctional protein that can act as an anchor for cell membrane cadherins (see
Chapter 3) or as a nuclear transcription factor (see Chapter 6). In
Xenopus
embryos, β-catenin begins to
accumulate in the dorsal region of the egg during the cytoplasmic movements of fertilization. β-catenin
continues to accumulate preferentially at the dorsal side throughout early cleavage, and this
accumulation is seen in the nuclei of the dorsal cells (
Figure 10.23A-
D;
Schneider et al. 1996;
Larabell
et al. 1997). This region of β-catenin accumulation originally appears to cover both the Nieuwkoop
center and organizer regions. During later cleavage, the cells containing β-catenin may reside
specifically in the Nieuwkoop center (
Heasman et al. 1994a;
Guger and Gumbiner 1995).
Figure 10.23
The role of Wnt pathway proteins in dorsal-ventral axis
specification. (A-D) Differential translocation of β-catenin into
Xenopus
blastomere nuclei. (A) Early 2-cell stage of
Xenopus
,
showing β-catenin (orange) predominantly at the dorsal
(more...)
β-catenin is necessary for forming the dorsal axis, since experimental depletion of β-catenin transcripts
with antisense oligonucleotides results in the lack of dorsal structures (
Heasman et al. 1994a).
Moreover, the injection of exogenous β-catenin into the ventral side of the embryo produces a
secondary axis (
Funayama et al. 1995;
Guger and Gumbiner 1995). β-catenin is part of the Wnt signal
transduction pathway and is negatively regulated by the glycogen synthase kinase 3 (GSK-3; see
Chapter 6). GSK-3 also plays a critical role in axis formation by suppressing dorsal fates. Activated
GSK-3 blocks axis formation when added to the egg (
Pierce and Kimelman 1995;
He et al. 1995;
Yost
et al. 1996). If endogenous GSK-3 is knocked out by a dominant negative protein in the ventral cells of
the early embryo, a second axis forms (
Figure 10.23E).
So how can β-catenin become localized to the future dorsal cells of the blastula? Labeling experiments
(
Yost et al. 1996;
Larabell et al. 1997) suggest that β-catenin is initially synthesized (from maternal
Your preview ends here
Eager to read complete document? Join bartleby learn and gain access to the full version
- Access to all documents
- Unlimited textbook solutions
- 24/7 expert homework help
Related Questions
This is one given problem on my homework assignment for developmental biology. Thank you for the help!Describe the effects of FGF and RA on each other in limb bud development. Give a specific example of their effects on each other in this capacity. Knowing this, propose a question/hypothesis to investigate the mechanism of how a specific morphogen controls pattern if you know that the morphogen is both necessary and sufficient AND is expressed in the correct spatial/temporal frame.
arrow_forward
Considering that you manipulated a zebrafish embryo by injecting high levels of retinoic acid so that no anterior genes will be expressed. Surprisingly, after embryonic development, you are still able to observed the presence of anterior structures.
What could explain this observation, does this mean any errors has been committes( provide a specific answer or postulatiom of why it happened)?
What changes in your experiment would you make to achieve your objective?
arrow_forward
The nematode C. elegans has proved to be a valuable model organism for studies of cell birth, cell asymmetry, and cell death. What properties of C. elegans render it so well suited for these studies? Why is so much information from C. elegans experiments of use to investigators interested in mammalian development?
arrow_forward
To explain: one or more possible explanations for
the slower rate of evolutionary change in the human
lineage versus the rat lineage.
arrow_forward
For the first experiment ever on Drosophila mutations. Answer the following questions.
a. What is the title of the first published paper explained the experiment and what is the name of the Author?
b. What is the first mutation discovered in Drosophila?
c. Explain the changes in the Drosophila yellow mutant (Y)compared to wild type.
arrow_forward
Figure 13-7 illustrates the expression of the Ultrabithorax(Ubx) Hox protein in developing flight appendages.What is the relationship between where the protein isexpressed and the phenotype resulting from the loss ofits expression (shown in Figure 13-1)?
arrow_forward
please give answer for all parts .
asap
arrow_forward
WRT is a gene in C. elegans worms which is normally expressed in a specific stage of development.
Worms without this gene (wrt-/wrt- genotype) survive, but grow too large, never develop some
parts of their anatomy. Over-expression of WRT results in a different phenotype: the worms have
stunted growth, developing mature anatomical structures too early in their growth. The following
experiments were used to learn how WRT is regulated.
a.
Several genetic loci that are also involved in development in the worm are suspected of being
regulators of WRT. Mating experiments shows that some of these genes have epistatic
relationships with WRT, and others don't. Which are likely to be WRT regulators?
i. Genes epistatic to WRT are unlikely to regulate WRT
ii. Genes epistatic to WRT are likely to regulate WRT
iii. Epistasis cannot tell us whether another gene is in the same pathway as WRT
b. Deletion of locus 1 in a WRT wildtype background causes the wrt- associated phenotype (large
but…
arrow_forward
Define Modularity and Combinatorial control and give an example of both in developmental biology. Please make your definitions in-depth
arrow_forward
Using the information in the Reading file part II and textbook, answer the following questions:
Whales possess vestigial organs in the form of pelvic girdle and hind limb bones. How would you explain this?
How can you explain that, while forelimbs of bats and human arms are used for vastly different purposes, they do have similar anatomy?
PART III. BIOINFORMATICS AND GENE MODIFICATION EVIDENCE
SICKLE CELL ANEMIA: GENETICS AND EVOLUTIONCircle the mutation in DNA of the sickle-cell beta-globin gene fragment in the table below:
Wild-type beta-globin gene fragment
C A C G T A G A C T G A G G A C T C
Sickle-cell beta-globin gene fragment
C A C G T A G A C T G A G G A C A C
Transcribe beta-globin DNA into mRNA. Note: DNA is already arranged in triplet sets (below).
Translate mRNA codons into amino acids of hemoglobin protein sequence using the chart on page 3.
Wild-type…
arrow_forward
Another way to study the role of proteins (e.g., transcription factors) that function in development is to microinject the mRNA that encodes a protein, or the purified protein itself, into an oocyte or embryo, and then determine how this affects the subsequent development of the embryo, larva, and adult. For example, if Bicoid protein is injected into the posterior region of an oocyte, the resulting embryo will develop into a larva that has anterior structures at both ends. Based on your understanding of the function of each developmental gene, what would be the predicted phenotype if the following proteins or mRNAs were injected into normal oocytes?
A. Nanos mRNA injected into the anterior end of an oocyte
B. Antp protein injected into the posterior end of an embryo
C. Toll mRNA injected into the dorsal side of an early embryo
arrow_forward
The homeotic mutation Antennapedia causes mutant Drosophila to have legs in place of antennae and is a dominant gain of-function mutation. What are the properties of such mutations?How does the Antennapedia gene change antennae into legs?
arrow_forward
You have identified a Drosophila gene that is expressed exclusively in the odd-numbered "stripes" in the cellular blastoderm. Assuming that this gene is not redundant, what
would be the most likely phenotype cause by a loss-of-function mutation in this gene?
an embryo missing odd numbered segments
an adult fly with a second pair of wings instead of halteres
an embryo with two anterior ends
an embryo missing even-numbered segments
an embryo missing larval segments 3-10
arrow_forward
SUBJECT: Developmental Biology
A. CRANIAL (CEPHALIC) NEURAL CREST
B. TRUNK NEURAL CREST
C. VAGAL AND SACRAL NEURAL CREST
Trace their differentiation pathways from the time the precursor cells break away from the neural tube.
Make sure that this three (3) derivatives utilize different migratory pathways.
arrow_forward
(If anyone can please help me, I need a short explanation of why the answer to each question is correct)
1. The evolution/development of the skeletal system found in the Deuterostomes, is made up of two molecules, Ca+2PO4-2 and collagen These molecules are produced released from by a specialized type of stem cell. Therefore, one would expect that these stem cells perform which of the following a) the storage and the release of Ca+2PO4-2 and collagen from vesicles from the Golgi apparatus, b) high levels of protein synthesis c) active transport of amino acids and ions into the stem cells through active transport, d) oxidation of pyruvate by the mitochondria, e) all are correct.
2. As a unicellular eukaryote, the Amoeba is an important example of which of the following? a) It reacts to a stimulus. b) when it reacts , the stimulus produces an electrical impulse that causes a change in other proteins. c) It generates motor output (movement). d) It uses specific membrane embedded…
arrow_forward
A reduced or incompletely developed structure in an organism that has no (or reduced) function (hint - not looking for an example of this, but rather the term)
arrow_forward
The FOXP2 gene is associated with speech in humans. It is also found in chimpanzees, gorillas, orangutans, rhesus macaques, and even the mouse, yet none of these mammals speak. Develop a hypothesis that explains why FOXP2 supports speech in humans but not in other mammals.
arrow_forward
please answer this two questions ..
and give answer asap
arrow_forward
The Drosophila homeotic mutation spineless aristapedia (ssa ) results in the formation of a miniature tarsal structure (normally part of the leg) on the end of the antenna. What insight is provided by (ssa ) concerning the role of genes during determination?
arrow_forward
(Please help me with all of these, I need a short explanation as to why it is the answer for each question)...
1. The evolution/development of the skeletal system found in the Deuterostomes, is made up of two molecules, Ca+2PO4-2 and collagen These molecules are produced released from by a specialized type of stem cell. Therefore, one would expect that these stem cells perform which of the following a) the storage and the release of Ca+2PO4-2 and collagen from vesicles from the Golgi apparatus, b) high levels of protein synthesis c) active transport of amino acids and ions into the stem cells through active transport, d) oxidation of pyruvate by the mitochondria, e) all are correct.
2. A multicellular eukaryote cell typically has enough available ATP to meet its needs for about 90 seconds. What would cause an active cell to exhaust its ATP supply so quickly? A) regular rest is a cellular requirement. B) Photosynthesis used up all ATP. C) ATP is transported into other cells. D)…
arrow_forward
Need help fast
Contrast ST and NST in humans and non-human animals. Why does Marchand (or any other evidence) conclude it probably doesn’t happen in adult humans, and what new techniques show it is present in some adult humans .
arrow_forward
The MyoD gene in mammals plays a role in skeletal muscle-cell differentiation, whereas the Hox genes are homeotic genes that play a role in the differentiation of particular regions of the body. Explain how the functions of these genes are similar and different.
arrow_forward
The three homeodomain proteins Abd-B, Abd-A, andUbx are encoded by genes within the Bithorax complexof Drosophila. In wild-type embryos, the Abd-B gene isexpressed in the posterior abdominal segments, Abd-Ain the middle abdominal segments, and Ubx in the anterior abdominal and posterior thoracic segments. Whenthe Abd-B gene is deleted, Abd-A is expressed in both themiddle and the posterior abdominal segments. WhenAbd-A is deleted, Ubx is expressed in the posterior thoraxand in the anterior and middle abdominal segments.When Ubx is deleted, the patterns of Abd-A and Abd-Bexpression are unchanged from wild type. When bothAbd-A and Abd-B are deleted, Ubx is expressed in all segments from the posterior thorax to the posterior end ofthe embryo. Explain these observations, taking into consideration the fact that the gap genes control the initialexpression patterns of the homeotic genes
arrow_forward
Background: In class we did a project about m and m’s and there ability to evolve and natural selection. (Yes I know they can’t actually evolve it was just used as a example in class) however I can’t figure out this question.
Occasionally a mutant may occur and produce a candy that is misshapen or Pointier or flatter than the rest. Often this proves to be a weakness, but a rare mutation may confer extra M&M durability. Other mutations in M&Ms could occur ( like ones that result in a new color). A mutant un you population represents a new _________.
What goes in the blank
arrow_forward
Chimpanzees and humans are almost 99% identical in terms of DNA sequence. However, we are very different to chimpanzees in many ways including appearance. What makes us so different?
Please relate in genetics lessons - Developmental Genes, Epigenetics
arrow_forward
Discuss the molecular players in myogenesis. Specify their role in the process.
arrow_forward
We know quite a bit about Notch/Delta and Hes gene oscillations during embryonic development. What do oscillations of these genes in the adult neural stem cell niche actually look like? How do these oscillations result in the progression from stem cell to neuron?
arrow_forward
Temperature-dependent sex determination (TSD) is the method of sex determination in sea turtles. In TSD, temperature experienced during embryonic development determines the sex of the offspring. TSD is only observed in reptiles and teleost fish. The molecular mechanisms are not well understood, but the production of estrogen, which causes female development, seems to be critical. A potential pathway is shown in Figure 1.
arrow_forward
SEE MORE QUESTIONS
Recommended textbooks for you
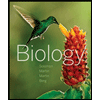
Biology (MindTap Course List)
Biology
ISBN:9781337392938
Author:Eldra Solomon, Charles Martin, Diana W. Martin, Linda R. Berg
Publisher:Cengage Learning
Related Questions
- This is one given problem on my homework assignment for developmental biology. Thank you for the help!Describe the effects of FGF and RA on each other in limb bud development. Give a specific example of their effects on each other in this capacity. Knowing this, propose a question/hypothesis to investigate the mechanism of how a specific morphogen controls pattern if you know that the morphogen is both necessary and sufficient AND is expressed in the correct spatial/temporal frame.arrow_forwardConsidering that you manipulated a zebrafish embryo by injecting high levels of retinoic acid so that no anterior genes will be expressed. Surprisingly, after embryonic development, you are still able to observed the presence of anterior structures. What could explain this observation, does this mean any errors has been committes( provide a specific answer or postulatiom of why it happened)? What changes in your experiment would you make to achieve your objective?arrow_forwardThe nematode C. elegans has proved to be a valuable model organism for studies of cell birth, cell asymmetry, and cell death. What properties of C. elegans render it so well suited for these studies? Why is so much information from C. elegans experiments of use to investigators interested in mammalian development?arrow_forward
- To explain: one or more possible explanations for the slower rate of evolutionary change in the human lineage versus the rat lineage.arrow_forwardFor the first experiment ever on Drosophila mutations. Answer the following questions. a. What is the title of the first published paper explained the experiment and what is the name of the Author? b. What is the first mutation discovered in Drosophila? c. Explain the changes in the Drosophila yellow mutant (Y)compared to wild type.arrow_forwardFigure 13-7 illustrates the expression of the Ultrabithorax(Ubx) Hox protein in developing flight appendages.What is the relationship between where the protein isexpressed and the phenotype resulting from the loss ofits expression (shown in Figure 13-1)?arrow_forward
- please give answer for all parts . asaparrow_forwardWRT is a gene in C. elegans worms which is normally expressed in a specific stage of development. Worms without this gene (wrt-/wrt- genotype) survive, but grow too large, never develop some parts of their anatomy. Over-expression of WRT results in a different phenotype: the worms have stunted growth, developing mature anatomical structures too early in their growth. The following experiments were used to learn how WRT is regulated. a. Several genetic loci that are also involved in development in the worm are suspected of being regulators of WRT. Mating experiments shows that some of these genes have epistatic relationships with WRT, and others don't. Which are likely to be WRT regulators? i. Genes epistatic to WRT are unlikely to regulate WRT ii. Genes epistatic to WRT are likely to regulate WRT iii. Epistasis cannot tell us whether another gene is in the same pathway as WRT b. Deletion of locus 1 in a WRT wildtype background causes the wrt- associated phenotype (large but…arrow_forwardDefine Modularity and Combinatorial control and give an example of both in developmental biology. Please make your definitions in-deptharrow_forward
- Using the information in the Reading file part II and textbook, answer the following questions: Whales possess vestigial organs in the form of pelvic girdle and hind limb bones. How would you explain this? How can you explain that, while forelimbs of bats and human arms are used for vastly different purposes, they do have similar anatomy? PART III. BIOINFORMATICS AND GENE MODIFICATION EVIDENCE SICKLE CELL ANEMIA: GENETICS AND EVOLUTIONCircle the mutation in DNA of the sickle-cell beta-globin gene fragment in the table below: Wild-type beta-globin gene fragment C A C G T A G A C T G A G G A C T C Sickle-cell beta-globin gene fragment C A C G T A G A C T G A G G A C A C Transcribe beta-globin DNA into mRNA. Note: DNA is already arranged in triplet sets (below). Translate mRNA codons into amino acids of hemoglobin protein sequence using the chart on page 3. Wild-type…arrow_forwardAnother way to study the role of proteins (e.g., transcription factors) that function in development is to microinject the mRNA that encodes a protein, or the purified protein itself, into an oocyte or embryo, and then determine how this affects the subsequent development of the embryo, larva, and adult. For example, if Bicoid protein is injected into the posterior region of an oocyte, the resulting embryo will develop into a larva that has anterior structures at both ends. Based on your understanding of the function of each developmental gene, what would be the predicted phenotype if the following proteins or mRNAs were injected into normal oocytes? A. Nanos mRNA injected into the anterior end of an oocyte B. Antp protein injected into the posterior end of an embryo C. Toll mRNA injected into the dorsal side of an early embryoarrow_forwardThe homeotic mutation Antennapedia causes mutant Drosophila to have legs in place of antennae and is a dominant gain of-function mutation. What are the properties of such mutations?How does the Antennapedia gene change antennae into legs?arrow_forward
arrow_back_ios
SEE MORE QUESTIONS
arrow_forward_ios
Recommended textbooks for you
- Biology (MindTap Course List)BiologyISBN:9781337392938Author:Eldra Solomon, Charles Martin, Diana W. Martin, Linda R. BergPublisher:Cengage Learning
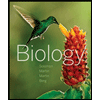
Biology (MindTap Course List)
Biology
ISBN:9781337392938
Author:Eldra Solomon, Charles Martin, Diana W. Martin, Linda R. Berg
Publisher:Cengage Learning
Browse Popular Homework Q&A
Q: Answer the following questions for the function
f(x)=sin2(x/6)
defined on the interval…
Q: State specifically the norm you intend to violate. Explain how you know this is a norm. ( for…
Q: Read the descriptions below of two substances and an experiment on each. Decide whether the result…
Q: Assuming that the crabmeat yield 25% meat. How many pound of whole live crab would you have to buy…
Q: 1 Consider AABC.
YA
8
-6
-8
4
A
--8--6-4-221416181
B
#
Paralelogram PQRS has verticas
PXC-4), and S…
Q: Inventory Control
Jesaki Publishing sells 50,000 copies of a certain book each year. It costs the…
Q: Calculate the accured interest in dollars, and the total proceeds in dollars of the bond sale round…
Q: This lab is about Secure Socket Layer (SSL).
For each of the first 8 Ethernet frames, specify the…
Q: The Campbell Company is considering adding a robotic paint sprayer to its production line. The…
Q: Carbon 14 has a half-life of 5730 years, calculate the activity due to C-14 in 1.00 kg of carbon.
Q: A 10 kg block is at rest on a frictionless 30° inclined plane. If a force of 60 N is applied
to the…
Q: The graph of fis shown in the figure. Sketch a graph of
the derivative of f.
K
5
5
4
3
2
+
-6-5 -4…
Q: will D and E be answered or will i be given my credit back
Q: return and standard deviation of Netflix (NFLX) stock were 3.5% and 10%, respectively. For the same…
Q: Protein kinase A is activated by:
phosphorylation of its catalytic subunit
phosphorylation of its…
Q: Find an equation of the tangent line to the curve at the given point.
xy + x^2y^2 = 20, (4,1)…
Q: Locate any relative extrema and inflection points of the function y:
-- Inx. Use a graphing utility…
Q: The work function for metallic Ni is 5 eV.
a) Calculate the threshold frequency of this material.…
Q: 6. Find the length of XY to the nearest hundredth:
Y
28°
5.1
Q: The price that watermelon farmers get for their watermelons has a normal distribution with unknown…
Q: Suppose that IQ scores have a bell-shaped distribution with a mean of 104 and a standard deviation…
Q: a. If charges flow very slowly through a metal, why does it not require several hours for a light to…
Q: If you have to push a 16 kg crate with a force of 32 N to get it to start sliding across the ground,…
Q: 47% of U.S. adults have very little confidence in newspapers. You randomly select 10 U.S. adults.…
Q: A cat leaps to catch a bird. If the cat's jump was at 60.0° off the ground and its initial velocity…